Chemistry of Materials, Magnetic & Electronic Phenomena
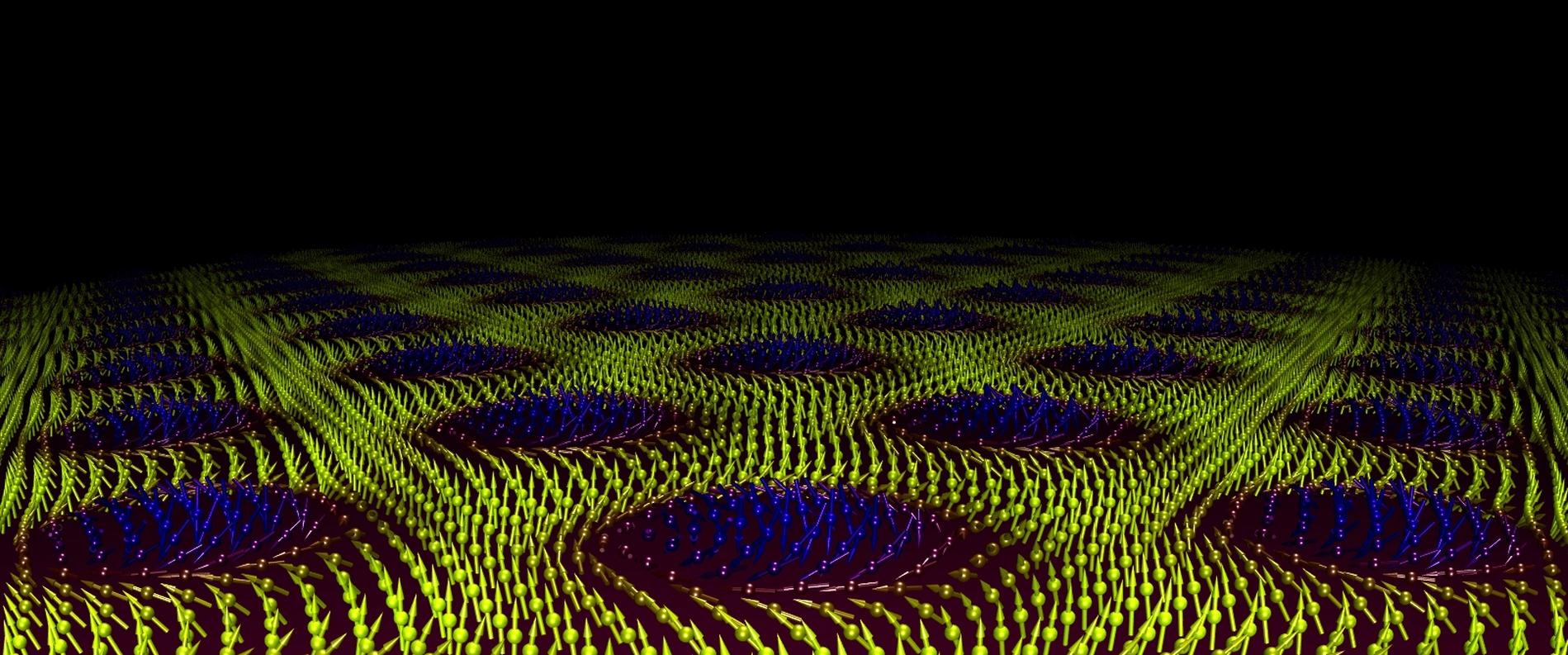
Modern technology is underpinned by the science of materials, from semiconductors and lasers to batteries and magnetic storage materials.
Read about future science
Better Superconductors to Improve Cancer DiagnosisClearly its composition is very important to the physical properties of a material, but so is the structure, on scales all the way down to a micro- or nanoscopic level. Completely new behaviour often appears in complex materials that would not be predicted from the properties of its components.
Neutrons are able to probe structures on length scales which range from the distance between atoms in a crystal to centimeters. The wave-like nature of the neutron as a quantum particle causes it to scatter from regularly spaced atoms in a crystal, producing interference patterns, which can be used to reconstruct crystal structures. Not only this, but neutrons are able to gain or lose energy as they interact, extracting even more information.
Neutrons also carry a magnetic moment, which allows us to use them to probe magnetic patterns inside materials. Examples range from switchable domains in the magnetic storage layer in hard disks, or spontaneous patterns of magnetisation inside exotic materials caused by subtle electronic interactions.
Current Science
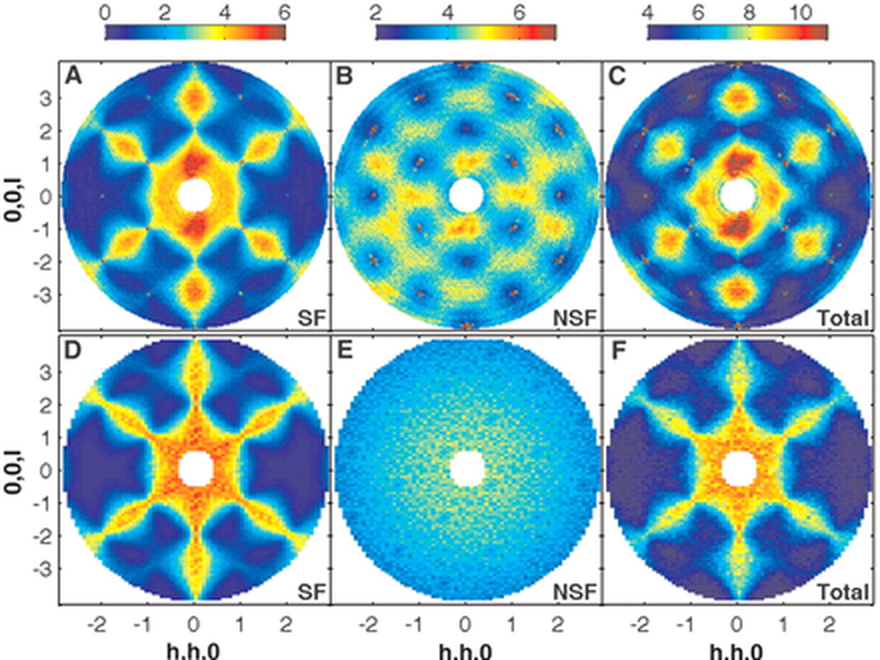
Magnetic monopoles are the magnetic equivalent of electrically charged particles. They are like an isolated north or south pole, without a matching partner of the other sign. Despite being theoretically possible they have never been observed, even after a great deal of searching over many decades.
In the artificial universe provided by the materials known as spin glasses it is possible to create arrangements of magnetic moments known as Dirac strings. The ends of these strings can be separated by long distances and behave completely independently in a way equivalent to monopoles. Due to their sensitivity to magnetism, neutrons are a powerful tool for investigating these phenomena (above).
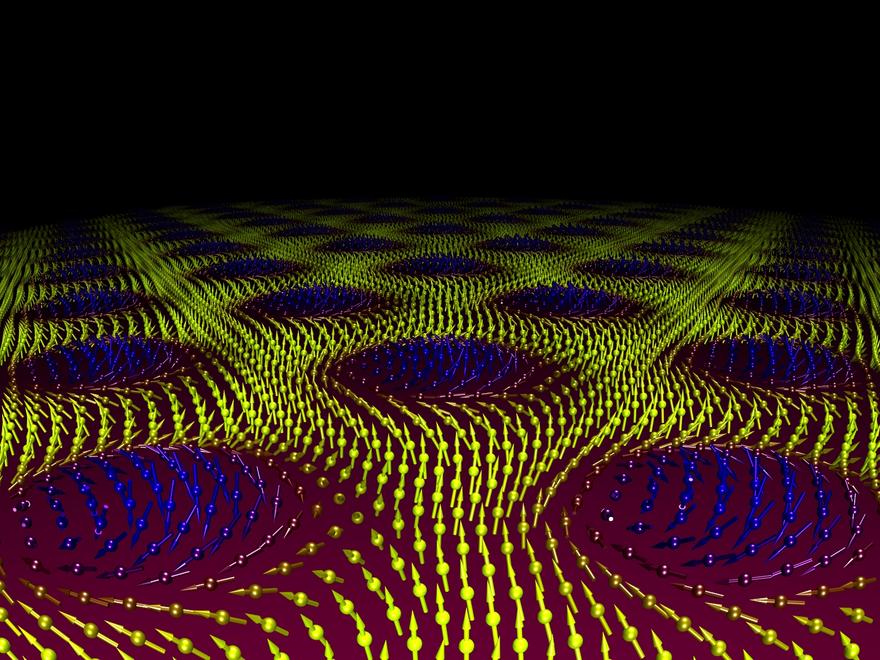
In 1962 the British theoretical physicist Tony Skyrme predicted the existence of a new particle. Unlike an electron or quark, this is a particle-like feature that exists within its own universe created by certain types of magnetic material. These so-called Skyrmions can move, interact, and annihilate with their own antiparticles in similar way to elementary particles. They are a type of topological soliton—like a knot on a rope which can be moved but not destroyed without cutting the rope itself.
The unique properties of neutrons allow us to view the patterns formed by Skyrmions when they form lattices under certain conditions of temperature and magnetic field (above). Pioneering work at ESS partner institute the Heinz Meier-Leibnitz Zentrum in Garching, Germany first demonstrated the existence of these particles in 2009 using small angle neutron scattering (SANS), one of the techniques that will be available at ESS on the SKADI instrument.
These fascinating particles show great promise for future magnetic storage technologies, and neutrons are a key method for exploring their properties.
Further reading:
Skyrmions: a twisted future. Physics World, May 2017
S. Mühlbauer et al. Science 13, 915-919 (2009)
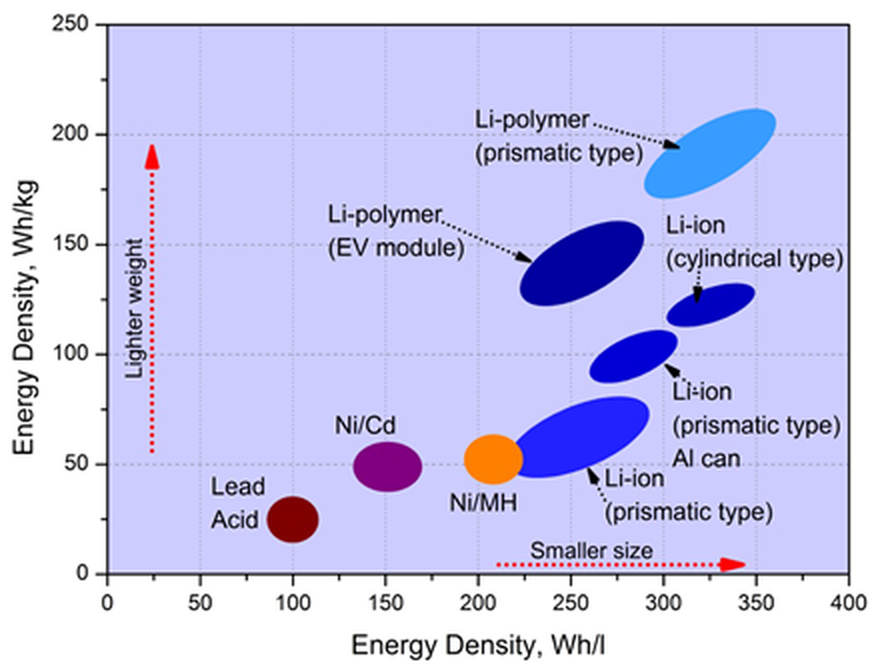
Rechargeable batteries currently power a wide range of products, including power and emergency storage systems, hybrid electric vehicles (HEV) and other EVs as well as the ubiquitous portable electronic devices found in our daily lives. As part of long-term sustainable energy storage solutions, further research efforts to improve performance and stability are crucial, particularly to monitor performance and failure mechanisms of batteries in operando under a range of environmental conditions.
Commercial lithium-ion batteries are being studied using electrochemical, diffraction and radiography techniques in operando as a function of temperature and charge-discharge rate. Neutron powder diffraction allowed the charge-discharge processes to be investigated at the cathode and anode and radiography gave insights into the macro-structural deformations and lithium redistribution within the battery as a function of state of charge and current density.
Despite remarkable progress, lithium ion batteries with higher energy density and cycle life are required for next generation consumer electronics. Work in this area includes looking at other anode materials, such as silicon based alloys that offer higher discharge capacity but remain challenging due to material degradation during extended cycling.
Further reading:
Science Drivers

Every major step in the development of human civilization has been marked by advances in materials and human understanding of them. Discovering where atoms are and what they do are key to understanding and tailoring properties. The ESS, with its unparalleled brightness and innovative instrument suite, will be an indispensable tool to make breakthroughs in materials research.
This field is multidisciplinary stretching across energy, chemical, environmental and life science as well as electronics and geoscience. The grand goal that unites these disparate fields is the desire to understand and impart functionality to materials. Society needs better catalysts, improved construction materials and safer pharmaceuticals and human demands on materials drive their increasing complexity. What is required in modern materials research is the determination of structure and dynamics of real world materials under real world conditions. The deep penetrating power of the neutron allows researchers to peer into the material itself while it is in action, yielding insights that can not be obtained by other means. The high brilliance of ESS will allow researchers to view decreasing gauge volumes inside devices, gaining insight into physical and chemical processes in real time. The ever increasing complexity and nano-structuring of functional materials will be studied with greater precision and in more dilute real world forms. These insights have led to the expert design and tailoring of materials for a variety of applications that benefit society. For an advanced understanding of physical and chemical properties, scientists will be able to harness the combined power of advances in experimental, theoretical and computational methods available at ESS. The key experimental techniques are diffraction, small angle neutron scattering and imaging for structure as well as inelastic neutron scattering, quasi-elastic neutron scattering and spin-echo for dynamics.
Structure and in-situ processing
Crystallography is the cornerstone of materials characterisation, determining where atoms are in space. Without this knowledge, it would be impossible to relate structure to properties. Neutron scattering is highly complementary to X-ray scattering and, ideally, requires flexible instrument set-up (resolution, Q-range and flux), which is inherent to the ESS long pulse source. In-situ processing is an extension of crystallography -- investigation of a system in real-time as a function of changing variables, such as time, temperature, pressure, magnetic field and/or chemical potential. It can also be applied to investigating the evolution of dynamics and changes in microstructure over time. Neutron scattering has barely scratched the surface of possibilities offered by high flux-, time- and spatial-resolution instruments. At ESS, scientists will perform multi-technique measurements and combine them with computational modeling methods, connecting theory and experiment to understand processes in unparalleled detail. The instrument suite reflects the various needs for structural investigation by providing a general-purpose polarized SANS, broad-band small sample SANS, thermal powder diffractometer, bispectral powder diffractometer, pulsed monochromatic powder diffractometer, materials science & engineering diffractometer, extreme conditions instrument, macromolecular diffractometer and the single crystal magnetism diffractometer.
Catalytically active materials
Catalysis, the ability of some substances to alter the rate of chemical reactions without being consumed, was recognized more than 150 years ago and has been applied on an industrial scale since the beginning of the last century. To understand catalytic activity, or to tailor a catalyst to do a specific job, it is necessary to know the individual steps in the catalytic process in detail. Many experimental techniques are deployed to understand and tailor these materials for their real world applications. The unique utility of neutrons resides in their ability to locate hydrogen atoms and determine their chemical state as this provides critical insight into the catalytic activity. While quenching a catalytic system in operando is often used to perform quasi-static neutron measurements of the frozen state, this method is of limited practical use as the system is not investigated in its active states. The ESS will facilitate routine, time-resolved measurements breaking new ground in fast catalytic processes at the gas-solid interface. Combining these measurements at ESS with computer simulations of model and real systems facilitated via the ESS-DMSC, has the potential to provide new insight into the structure and function of catalysts. Key instruments that address these needs are the thermal powder diffractometer, pulsed monochromatic powder diffractometer, bispectral powder diffractometer, cold chopper spectrometer, vibrational spectroscopy instrument and backscattering spectrometer.
Health and pharmaceuticals
The development of pharmaceuticals must proceed through several stages in order to ensure their safety and efficacy before their introduction into the market. Many problems that delay new drug approvals are related to insufficient understanding of the drug's physical properties and manufacturing processes. Although the intrinsic activity of an active pharmaceutical ingredient is determined by its chemical structure in solution and how it interacts with its targets (i.e. receptors and enzymes), the effectiveness of drug delivery depends largely on its physicochemical and materials properties in the solid state. It is critical to identify the degree of polymorphism and stability of the active ingredient in the presence of other polymorphs before development. Choosing the lowest free energy polymorph usually leads to dissolution problems and low bioavailability where as the highest energy polymorph may result in high solubility but accompanied by high toxicity and very short half life. Striking a balance between the free Gibbs energy of the active ingredient is crucially important as the consequences of incomplete evaluation can be disastrous; the lowest energy polymorph of the HIV protease inhibitor Ritonavir rendered inactive the bioactive higher energy polymorph.
Despite all the experimental tools available, the energy landscape of drug polymorphs is not exhaustively investigated during the discovery phase. Such a task is not straightforward and in most cases Raman scattering and powder X-ray diffraction are insufficient. The absence of selection rules and access to both spatial and time correlations is unique to neutron spectroscopy. Wider use of neutron scattering techniques has the potential to make a huge impact in this evaluation process. By combining neutron techniques with the existing arsenal of methodologies it will be possible to locate the hydrogen atoms in a more precise way and to understand their dynamics in molecular drugs, thus mapping the energy landscape more precisely and understanding polymorphism more accurately. For structural studies, ESS's higher flux and flexibility in tailoring the long pulse will be crucial to studying processes in-situ using the thermal powder diffractometer and the pulsed monochromatic powder diffractometer. Dynamics can be determined by neutron spectroscopy using the vibrational spectroscopy instrument, the backscattering spectrometer, the cold chopper spectrometer or the bispectral chopper spectrometer.
Environmental and green chemistry
Modeling of cloud formation by atmospheric aerosol droplets as well as safe and economical processes for water purification in developing countries are examples in environmental chemistry for which neutron investigations were essential. Investigating the environmental fate and the structure - activity relationship of engineered nanomaterials is essential to understand their environmental impact and to provide a framework for improved nanomaterials that are more environmentally benign. Only a few structure - activity relationships have been developed. In addition to SANS, the multi-purpose imaging station at the ESS will allow materials to be studied non-destructively and under operational conditions. Following processes in-situ will also play a fundamental role at the ESS in order to develop alternatives that have a lower environmental footprint. The high neutron flux of the ESS's horizontal reflectometer will make possible studies of interfacial reactions at the millisecond to second timescale, allowing the kinetics and mechanism of processes to be understood.
Waste management
In Europe, natural and engineered clay barriers are considered in the framework of the multi-barrier concept used for the disposal of high and long-life radioactive wastes at great depth while concrete, the world’s most versatile and most widely used material, is conventionally utilized as repository barriers for intermediate-level waste in many countries. The quality of these barriers and their resistance to water is critical in safeguarding the environment. The quality of hardened concrete is greatly influenced by the water confined in the cementitious materials and how it is transmitted through cracks and pores, while in the case of clays, the dynamical properties of water molecules in the interlayer spacing is responsible for a wide range of water activity. In both cases, environmental conditions can interfere with these barriers and influence their service life. Neutron scattering is an advantageous and unique technique for understanding how water transmission is affected by confinement in such waste management materials.
Quasi-elastic neutron techniques have provided information about the dynamics of water molecules and their mobility (see figure). SANS, with its sensitivity to nanometer structural features, has made it possible to look into pores and water adsorption, and neutron imaging revealed the pore size distribution and the extent of cracking. With the higher neutron brightness provided by ESS, SANS and neutron imagining techniques will be able to grasp with far better resolution the nuances of the hydration process of various waste management materials allowing for a better understanding of their structure. The ESS spectrometer suite will allow for a continuous study of the dynamics of the various water populations found in concrete and clays. The combination of these experiments with the computer modeling insights into the dynamics of water provided by the ESS-DMSC will lead to the development of better and more durable barriers for waste management.
Novel materials
A recent review article stated that superconducting materials are "still alluring but hard to predict... ''. This statement encapsulates the grand challenge in materials chemistry: even after centuries of research and development in experimental and theoretical methods, it remains difficult to predict novel properties of materials. While our society greatly benefits from novel materials exhibiting negative thermal expansion, new light-emitting compounds or novel high temperature superconductors, our ability to discover them largely relies on scientific experience and intuition. In recent years, the chemical processes for forming new exciting and potentially technologically important compounds have become ever more extreme conditions in terms of pressure and temperature. This leads to smaller and more complex samples whose structure and chemistry must be understood.
Neutrons and X-rays are the most basic tools for characterizing these new materials. While X-rays often provide highly accurate information on complex symmetry, neutrons are best suited for accurately finding the position of atoms. These tools are indispensable for advancing understanding of novel materials and their properties. Often these advances in chemistry have proved resistant to the important information that neutrons can deliver. The unique capabilities of ESS will make possible a suite of diffractometers that will be able to measure ever smaller and more complex novel materials under real processing conditions, critically accelerating materials discovery. The dedicated cold crystal-analyser spectrometer and the extreme conditions instrument will be well suited to provide extreme environments with focusing optics for small samples and restrictive sample environments.

In recent years huge leaps in technological advances have been made by better understanding the electronic properties of materials.
Two strong fields of research within this domain are magnetism and superconductivity, long thought to be antagonistic properties. Interestingly, in innovative materials they are manifestations of a new quantum superposed ground state. This reveals the dominant trend in condensed matter research towards towards increasing complexity, both in the materials themselves as well as the theory to explain their phenomena. Neutrons investigate electronic properties via the interaction of its spin with the electronic state of condensed matter. The strength of scattering techniques lies in the ability to measure simultaneously spatial and time correlations, thus providing information on the different length and energy scales responsible for electronic phenomena. Such direct microscopic information makes neutron scattering ideal to benchmark experimental results against theoretical models.
Read about future science
Better Superconductors to Improve Cancer DiagnosisA few select examples are given below to support the wealth and breadth of neutron scattering and to illustrate the requirements for the future.
Thin film heterostructures based on metal oxide materials can display a broad range of physical phenomena including large spin polarisation, colossal magnetoresistance, electronic phase ordering, charge, orbital and spin ordering [1,2]. Thin films structure comprising oxide heterostructures are therefore envisaged to give rise to novel concepts in electronic devices. Polarised neutron reflectometry remains the only technique that can probe the internal magnetic structure of thin films, often different to their bulk counterparts due to surface effects. The gain in flux at ESS, combined with adequate instrumental options and sample environment, will enable reflectometers to routinely offer the capability to measure smaller samples and signals in addition to dynamic behaviour.
Magnetic domain structures, such as periodic antidot arrays, can be observed by grazing incidence scattering or small angle neutron scattering. Periodic antidot arrays, consisting of non-magnetic holes in a continuous magnetic film, have a great potential advantage over magnetic dots for data storage [3]. Such domains can be mapped using tomography in which one is able to visualise 3-dimensional magnetic domains (0.1 - mm sized) using phase contrast techniques [4]. However, polarised neutron scattering with micro-focused neutron beams from ordered nanometer sized magnetic domains on such antidot arrays are also of great potential for detecting local versus global structures and scanning lateral inhomogeneities.
Spin-lattice coupling is at the origin of many cross-correlation phenomena. An example is the frustrated CuFeO2 in which the application of short pulses of terahertz radiation, a dynamical coupling between spin and the electric dipole stabilises a collinear magnetic state by strong spin-lattice coupling [10]. To understand these cross-correlation phenomena magnetic exchange interactions must be quantified thus requiring neutron scattering with in-situ external perturbations to probe the resultant electromagnon. Further examples are those in multiferroic systems in which simultaneous crossed electric and magnetic fields are required to probe the phononic and magnetic components of the critical ferroelectric phase [11]. Of particular importance for future instrumentation will be low Q capabilities on all wide mapping diffraction and spectroscopic instruments with polarisation analysis capabilities and high Q and E resolution to probe the fine structure of such resultant electromagnons.
Emergent complex phenomena, such as fundamental magnetic and superconducting order, are studied on a microscopic level using diffraction and spectroscopic techniques. Such phenomena can be classed as more fundamental behaviour, in comparison to the more applied thin film heterostuctures , and as such it is hoped that these phenomena will become the basis for innovative technologies in the future. Indeed, one can be directed to the studies of flux-line lattices of practical, type II, superconductors, in which the effects of an applied magnetic fields are of crucial importance in trying to increase the efficiencies of materials that are used in high-field magnets, such as nmr, mri and particle-accelerator electromagnets. In the case of the unresolved nature of high temperature superconductivity, parametric studies, using both neutron diffraction and spectroscopy, are required to elucidate the subtle features of the superconducting phases related to the symmetries and energy scales involved [5]. Probing the excitation spectrum has been simplified recently with the possibility to measure four dimensional (S(Q,E) maps using time of flight spectrometers with position sensitive detectors that were not available before. The future for such instrumentation lies in greater signal to noise and polarisation analysis to elucidate the unaccounted-for spectral weight required for a comprehensive theory of high temperature superconductivity. Equally, polarisation analysis and greater signal to noise is particularly relevant for the study of higher order exchange interactions (quadrupolar, octopolar and anapolar) [7] in molecular magnets and f-electron compounds in which the weak exchange interactions places these phenomena at the forefront of technological feasibility.
Quantum critical behaviour can be accessed by tuning a phase transition to T = 0 K via pressure, magnetic field or doping (chemical pressure). Quantum fluctuations give rise to an abrupt change in the ground state when their energy scales (E =1 meV = 11.56 K = 10 T for 1µB) exceeds those of thermal fluctuations. The scattering profiles obtained tend to be broad and as the quantum critical point is approached the signal is weak [8]. A key requirement is to map out the inelastic response over a wide range of momentum and energy space as a function of temperature concomitant with magnetic field or external pressure. These restrictive sample environments requires small sample volumes. In addition, it is necessary to synthesise samples with homogenous doping that tends to leading to small sizes. As such, a dedicated extreme environment instrument is required with focusing optics for small samples and restrictive scattering geometries.
Fractional excitations further reveal the importance of wide mapping instrumentation. These play as an important role in strongly correlated behaviour. In particular the 1-D Heisenberg antiferromagnetic chain has proved fertile ground for the interplay between theory and neutron scattering by recently revealing a four-spinon continuum at high energies, which emerges when long range magnetic order is destroyed via quantum fluctuations [9]. The question remains whether fractional excitations are generic to low dimensional systems. In order to answer this polarisation analysis is required to separate the phonon from the magnetic contributions resulting in absolute units of the magnetic scattering.
Magnetic frustration and spin glasses display low frequency spin dynamics. These are typically probed by cold neutrons on chopper spectrometers, backscattering instruments and via the Fourier time of a spin echo instrument. The dynamics in these compounds are subtle in their differences. Wider Fourier times, coupled with greater flux, are required to dissociate relaxational dynamics from the signature of a resonating valence bond or the excitations of a magnetic monopole [13,14] out of a degenerate ground state manifold [15].
The role of topology in magnetism has revealed a number of exciting new magnetic states. One of these is the skyrmion lattice, see above, originating from a charged topological spin texture due to strong electronic correlations. Neutron scattering was able to reveal this most complex order to be a chiral lattice of topological particles [15]. Although chirality and topology are old concepts, in theoretical terms they are being revealed in an ever growing number of compounds. The study of such complex behaviour requires all the experimental attributes that have been mentioned thus far; polarisation analysis to separate chiral terms, high signal to noise for weak scattering features and small samples, absolute units of magnetic scattering to directly link theory with experiment.
Finally, the study of non-equilibrium phenomena in quantum many body systems has recently been opened due to developments in experimental technology. Pump probe effects can access dissipative behaviour that must be considered when providing a description of spin dynamics. An interesting example is the disappearance of the Kondo resonance upon the application of microwave radiation on a quantum dot, providing an on/off switch for the exchange interactions in Kondo systems [16]. In-situ measurements such as these do not require a drastic change in instrumentation, but the experimental requirements when applying microwave radiation, electric fields, and lasers must be considered carefully. It is generally accepted that non-equilibrium states will pave the way for novel scientific phenomena.
Clearly, neutron scattering will remain a powerful tool to probe spin correlations. Current theories rely on subtle details of these absolute neutron scattering cross sections and therefore it is also important to note that the beam profile, line-shape and ease-of-use are equally, or even more, important than raw flux. As such one expects, and will require, spherical polarimetry, polarisation analysis, phase contrast imaging and greater use of focusing optics to become routine in the exploration of spin correlations and electron-lattice interactions.
References
[1] H Zabel and K Theis-Brühl; J. Phys.: Condens. Matter 2003
[2] C A F Vaz; J. Phys.: Condens. Matter 24 333201 (Review article) 2012
[3] D. R. Lee, G. Srajer, M. R. Fitzsimmons, V. Metlushko and S. K. Sinha; Appl. Phys. Lett. 82, 82 (2003). DOI: 10.1063/1.1534919
[4] N. Kardjilov et al.; Nature Physics 4(5) , 399, (2008).
[5]J. M. Tranquada, P. M. Gehring, G. Shirane, S. Shamoto, and M. Sato, Phys. Rev. B 46, 5561-5575 (1992)
[6] Phys. Rev. Lett. 106, 057004 (2011) [4 pages]
[7] Magnani, N. and Carretta, S. and Caciuffo, R. and Santini, P. and Amoretti, G. and Hiess, A. and Rebizant, J. and Lander, G. H.; Phys. Rev. B, 78, 2008
[8] Hilbert v. L√∂hneysen, Achim Rosch, Matthias Vojta, and Peter Wölfle; Rev. Mod. Phys. 79, 1015‚ 2007
[9] M. Enderle, B. F√•k, H.-J. Mikeska, R. K. Kremer, A. Prokofiev, and W. Assmus; Phys. Rev. Lett. 104, 237207‚ 2010
[10] Nakajima, Taro and Suno, Azusa and Mitsuda, Setsuo and Terada, Noriki and Kimura, Shojiro and Kaneko, Koji and Yamauchi, Hiroki Phys. Rev. B 84,184401 (2011)
[11]Peter Lunkenheimer, Jens Müller, Stephan Krohns, Florian Schrettle, Alois Loidl, Benedikt Hartmann, Robert Rommel, Mariano de Souza, Chisa Hotta, John A. Schlueter & Michael Lang; Nature Materials 11, 755‚ 758 (2012) doi:10.1038/nmat3400.
[12] P. W. Anderson (1973). Mater. Res. Bull. 8 (2). doi:10.1016/0025-5408(73)90167-0.
[13] T. Fennell, P. P. Deen, A. R. Wildes, K. Schmalzl, D. Prabhakaran, A. T. Boothroyd, R. J. Aldus, D. F. McMorrow, S. T. Bramwell; Science 16 October 2009: Vol. 326 no. 5951 pp. 415-417
[14] C. Castelnovo, R. Moessner & S. L. Sondhi, Nature 451, 42-45 (3 January 2008)
[15] S. Mühlbauer, B. Binz, F. Jonietz, C. Pfleiderer, A. Rosch, A. Neubauer, R. Georgii, P. Böni; Science, 2009, Vol 323, Issue 5916
[16]J.M. Elzerman, S. De Franceschi, D. Goldhaber-Gordon, W.G. van der Wiel and L. Kouwenhoven, J. Low Temp. Phys. 118 (2000), 375.

Energy Materials are an integral part of our daily lives, with applications ranging from consumer electronics (battery life, size and power) to the drive to reduce our dependence on fossil fuels with alternatives such as solar- and fuel-cells to power vehicles and generators.
The properties of the neutron are ideally matched to investigate structural and dynamical processes, relating form with function. For example, the high penetration depth of the neutron can be used to probe complex systems and assemblies under working conditions. In many cases, the presence of light atoms such as oxygen, lithium and hydrogen require neutron-based techniques to elucidate structure and to determine mechanistic pathways. Fundamental research is also important to probe structure and dynamics in matter to understand and tailor physical properties so that next-generation materials can be designed to replace those used today.
Several representative examples are given below to highlight the current role of neutron scattering techniques.
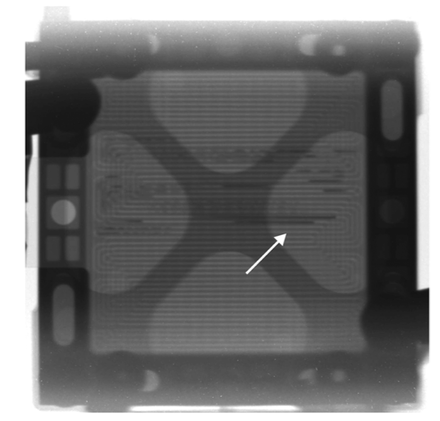
Fuel cell research covers fundamental studies on new electrode materials, electrolytes and membranes for solid-oxide fuel-cells (SOFC), proton exchange membrane fuel-cells (PEMFC) and proton ceramic fuel-cells (PCFC) up to monitoring the in operando performance and lifetime of membrane-electrode assemblies (MEA) and operating fuel-cell stacks. Neutron scattering contributions include diffraction studies to locate hydrogen [1] or vacancies in candidate materials, [2] SANS to follow the kinetics of water uptake in PEMFC electrolyte materials, [3] tomography to show the accumulation of water in gas-flow channels in an operating fuel PEMFC (see Figure), [4] QENS to probe molecular motions in ionomer membranes [5] and NSE to investigate proton dynamics in proton conducting perovskites. [6] The impact of neutron scattering in proton conducting oxides for fuel-cell research is also the topic of a recent review. [7]
Storage materials are a broad class of materials that include hydrides, hydrates, clathrates, frameworks, metal-organic frameworks (MOF) and nanotubes, with an emphasis on reversible hydrogen storage, encapsulation of other small molecules, such as alkanes, and trapping of harmful gases, such as carbon dioxide. Storing hydrogen safely, efficiently and reversibly is one of the main technological challenges before hydrogen can be adopted as a widespread energy carrier. In-situ neutron diffraction has been widely used to monitor hydrogenation/dehydrogenation reactions and site preferences. [8-10] SANS and USANS are powerful techniques to study the effects of small molecules confined in gas reservoirs, such as shale gases in coal, with implications for CO2 sequestration. [11-12] Unique information can be obtained from INS [13-15], QENS [16] and NVS [17-18] to quantify the amount of hydrogen in a sample and show if it is present as H2, by measuring the characteristic rotational spectrum of the hydrogen molecule. Understanding how clathrates form, coexist, transform and decompose [19-20] under particular conditions of temperature and pressure are crucial to their uses as fuel sources and to investigate the origins of pipeline blocking due to the formation of clathrate plugs. Also of interest is CO2 sequestration and understanding the possible implications of climate change on uncontrolled release of methane and CO2 from undersea clathrate beds.
Battery materials containing lithium are extensively used in applications, from consumer electronics to communications devices, and are increasingly replacing technology based on metal hydrides. Batteries rely on ion exchange and/or ionic conductivity properties of lithium and hydrogen and their properties are ideal for investigations using neutron scattering methods. Diffraction methods are widely used to study new materials and, increasingly, to probe battery materials in operando. [21-22] The field is of such high technological importance that a dedicated instrument (SPICA) was funded for structural investigations on battery materials, currently under commissioning, at the J-PARC spallation neutron source in Japan. Radiography also offers the opportunity to visualise working batteries under charge and discharge conditions. [23-24]
Thermoelectrics have the potential to revolutionise waste-heat recovery and the refrigeration industry. The challenge is to combine low thermal conductivity with a high electrical conductivity – the so-called ‘phonon glass-electron crystal’ concept. In addition to the many diffraction studies, INS is a key technique used to study the interplay of the rattler modes of guest molecules in the host materials with the acoustic phonon branches [25-26] that are responsible for thermal conductivity. INS data and computational calculations reveal that local soft and strongly anharmonic modes of antimony dimers dominate and drive the dynamic response, leading to the required low thermal conductivity.
Other materials, such as solar cells, based on thin film ceramics or organic semiconductors offer the possibility of generating low-cost energy from sunlight. Diffraction is widely used in this field to characterise the bulk structural properties of materials and to study materials with enhanced efficiency. [27] Neutron reflectometry offers the possibility to study interfaces in thin film blends and to monitor time dependent composition. [28] Neutron scattering investigations may also have an indirect impact. INS has recently been used to study quantum oscillations of nitrogen atoms in uranium nitride, [29] which could have implications in the design of generation IV nuclear reactors using uranium nitride as a fuel.
Energy materials require the full breadth of neutron scattering techniques to understand and optimise their properties. The combination of advanced instrumentation with flexible data acquisition modes, sample environment, data visualisation and data analysis offered by the ESS will provide unparalleled opportunities and insights for researchers in this field. Studies of new materials and processes necessarily begin with small sample volumes, accentuating the need to improve the performance and efficiency of neutron scattering instruments.
References
[1] I. Ahmed, C.S. Knee, M. Karlsson, S.G. Eriksson, P.F. Henry, A. Matic, D. Engberg, L. Börjesson. J. Alloys Compounds 2008, 450, 103-110.
[2] A. Magraso, J. M. Polfus, C. Frontera, J. Canales-Vasquez, L-E. Kalland, C.H. Hervoches, S. Erdal, R. Hancke, T. Norby, R. Haugsrud. J. Mater. Chem. 2012, 22(5), 1762-1764.
[3] G. Gebel, S. Lyonnard, H. Mendil-Jakani, A. Morin. J. Phys. Cond. Matt. 2011, 23(23), 234107.
[4] M. Strobl, I. Manke, N. Kardjilov, A. Hilger, M. Dawson, J. Banhart. J. Phys. D: Appl. Phys. 2009, 42, 243001.
[5] J-C. Perrin, S. Lyonnard, F. Volino. J. Phys. Chem. C 2007, 111, 3393.
[6] M.Karlsson, D. Engberg, M.E. Björketun, A. Matic, G.Wahnström, P.G.Sundell, P. Berastegui, I. Ahmed, P. Falus, B. Farago, L. Börjesson, S. Eriksson. Chem Mater. 2010, 22(3), 740-742.
[7] M. Karlsson. Dalton Trans. 2012, (in press).
[8] V.K. Peterson, Y. Liu, C.M. Brown, C.J. Kepert. J. Am. Chem. Soc. 2006, 128(49), 15578-9.
[9] T. Yildirim, M.R. Hartman. Phys. Rev. Lett. 2005, 95(21), 215504.
[10] Y. Yan, I. Telepeni, S.H. yang, X. Lin, W. Kockelmann, A. Dailly, A.J. Blake, W. Lewis, G.S. Walker, D.R. Allan, S.A. Barnett, N.R. Champness, M. Schroder. J. Am. Chem. Soc. 2010, 132(12), 4092.
[11] C. R. Clarkson, M. Freeman, L. He, M. Agamalian, Y. B. Melnichenko, M. Mastalerz, R. M. Bustin, A. P. Radli?A ski, T. P. Blach. Fuel 2012, 95(0), 371–385.
[12] Y. B. Melnichenko, L. He, R. Sakurovs, A. L. Kholodenko, T. Blach, M. Mastalerz, A. P. Radli?A ski, G. Cheng, D. F. R. Mildner. Fuel 2012, 91(1), 200–208.
[13] P.A. Georgiev, D.K. Ross, A. De Monte, U. Montaretto-Marullo, R.A.H. Edwards, A.J. Ramirez-Cuesta, M.A. Adams, D. Colognesi, Carbon 2005, 43(5), 895-906.
[14] A.J. Ramirez-Cuesta, P.C.H. Mitchell. Catalysis today 2007, 120(3-4), 368-373.
[15] C.M. Brown, Y. Liu, T. Yildirim, V.K. Peterson, C.J. Kepert. Nanotechnology 2009, 20(20), 204025.
[16] F. Salles, D.I. Kolokolov, H. Jobic, G. Maurin, P.L. Llewellyn, T. Devic, C. Serre, G. Ferey. J. Phys. Chem. C 2009, 113(18), 7802-7812.
[17] F. M. Mulder, B. Assfour, J. Huot, T. J. Dingemans, M. Wagemaker, A. J. Ramirez-Cuesta. J. Phys. Chem C 2010, 114(23), 10648-10655.
[18] L. Ulivi, M. Celli, A. Giannasi, A.J. Ramirez-Cuesta, M. Zoppi. J. Phys Cond. Matt. 2008, 20(10), 104242.
[19] M.M. Murshed, W.F. Kuhs. J. Phys. Chem. B 2009, 113(15), 5172-5180.
[20] D.K. Staykova, W.F. Kuhs, A.N. Salamatin, T. Hansen. J. Phys. Chem. B 2003, 107(37), 10299-10311.
[21] N. Sharma, V.K. Peterson, M.M. Elcombe, M. Avdeev, A.J. Studer, N. Blagojevic, R. Jusoff, N. Kamrulzaman. J. Power Sources 2010, 195, 8258-8266.
[22] S-I. Nishimura, G. Kobayashi, K. Ohoyama, R. Kanno, M. Yashima, A. Yamada. Nature Mat. 2008, 7, 707-711.
[23] N. Kardjilov, A. Hilger, I. Manke, M. Strobl, W. Treimer, J. Banhart. Nucl. Inst. Meth. A 2005, 542, 16-21.
[24] A. Senyshyn, M.J. Muehlbauer, K. Nikolowski, T. Pirling, H. Ehrenberg. J. Power Sources 2012, 203, 126-129.
[25] M. Christensen, A. B. Abrahansen, N.B. Chrsitensen, F. Juranyi, N. H. Andersen, K. Lefmann, J. Andreasson, C. R. H. Bahl, B. B. Iversen. Nature Mat. 2008. 7, 811-815.
[26] W. Schweika, R.P. Hermann, M. Prager, J. Persson, V. Keppens. Phys. Rev. Lett. 2007, 99, 125501.
[27] S. Schorr. Solar Energy Materials \& Solar Cells 2011, 95, 1482-1488.
[28] A. J. Parnell, A. D. F. Dunbar, A. J. Pearson, P. A. Staniec, A. J. C. Dennison, H. Hamamatsu, M. W. A. Skoda, D. G. Lidzey, R. A. L. Jones. Adv. Mater. 2010, 22, 2444-2447.
[29] A.A. Aczel, G.E. Granroth, G.J. MacDougall, W.J.L. Buyers, D.L. Abernathy, G.D. Samolyuk, G.M. Stocks, S.E. Nagler (submitted).